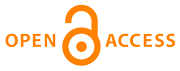 |
Home
>
Journal Issues
>
No 3 (2023) Technical mechanics
>
1
___________________________________________________
UDC 629.76
Technical mechanics, 2023, 3, 3 - 17
EFFECT OF THE SURFACE ROUGHNESS OF A POWER PLANT CHAMBER ON LOW-FREQUENCY SELF-OSCILLATIONS OF A COLD WORKING GAS
DOI:
https://doi.org/10.15407/itm2023.03.003
Nikolayev O. D., Bashliy I. D., Khoriak N. V., Bondarenko S. H.
Nikolayev O. D.
Institute of Technical Mechanics of the National Academy of Sciences of Ukraine and the State Space Agency of Ukraine
Bashliy I. D.
Institute of Technical Mechanics of the National Academy of Sciences of Ukraine and the State Space Agency of Ukraine
Khoriak N. V.
Institute of Technical Mechanics of the National Academy of Sciences of Ukraine and the State Space Agency of Ukraine
Bondarenko S. H.
Oles Honchar Dnipro National University
Dynamic processes in the combustion chamber have a significant effect on the characteristics of the working
processes of solid-propellant rocket engines (LPREs). Pressure jumps and a sharp increase in the local
temperature of the combustion products in non-stationary engine operation modes can lead to overrating
values of operating parameters and a failure of the LPRE combustion chamber structure. The dynamic processes
in the LPRE combustion chamber develop in a complex interconnection of a large number of physical and
chemical processes that occur in the gas-dynamic part of the working space of the engine chamber and often
lead to self-oscillating modes of engine operation. This is evidenced by numerous data on LPRE fire tests.
This paper presents the results of a numerical study of the effect of the LPRE chamber inner surface
roughness on LPRE operating parameter low-frequency self-oscillations. The study was made using up-to-date
computer simulation means and analysis.
Low-frequency (up to 1,000 Hz) oscillations in an LPRE combustion chamber were studied for a power plant test
chamber in cold operation with the use of two different approaches to numerical modeling of the dynamics
of in-chamber processes: the development and study of a 3D model of the dynamic system of combustion chamber
structure – combustion products using the finite element method and the development and study of an
axisymmetric 2D model of engine chamber gas flow using the finite volume method.
The study revealed a self-oscillatory flow regime caused by combustion product vorticity and acoustic feedback
due to vortices colliding with the chamber components or the LPRE nozzle. It was shown that accounting
for the wall roughness increased gas vorticity in the gas–solid dynamic interaction zone and the chamber
gas oscillation amplitude (on the average, by a factor of 2.5 at a maximum wall roughness height of 56 ?m).
The calculated gas flow pattern in the vorticity zones of the chamber and the low-frequency gas pressure
oscillation parameters are in qualitative agreement with the experimental ones.
solid propellant rocket engine, working process self-oscillations, combustion product flow vorticity,
acoustic oscillations, chamber wall surface roughness, finite volume method
1. Glazunov A.A., Eremin I.V., Zhiltsov K.N., Kostyushin K.V., Tyryshkin I.M., Shuvarikov V.A. Numerical investigation of the pressure pulsation magnitude and natural aeroacoustic frequencies in the combustion chambers with a charge of a complex shape. Tomsk State University Journal of Mathematics and Mechanics. 2018. No. 53. pp. 59?72.
https://doi.org/10.17223/19988621/53/6
2. Prisnyakov V. F. Solid-Propellant Rocket Engine Dynamics. Moscow: Mashinistroyeniye, 1984. 248 pp. (in Russian).
3. Fabignon Y., Dupays J., Avalon G., Vuillot F., Lupoglazoff N., Casalis G., Prevost M. Instabilities and pressure oscillations in solid rocket motors. Aerospace Science and Technology. 2003. V. 7. No. 3. Pp. 191-200.
https://doi.org/10.1016/S1270-9638(02)01194-X
4. Zeldovich J. B. Selected Works. Chemical Physics and Hydrodynamics. Moscow: Nauka, 1984. 374 pp.
5. Larionov V. M., Zaripov R. G. Gas Self-Oscillations in Plants with Combustion. Kazan: Kazan State Technical University, 2003. 228 pp. (in Russian).
6. Culick F.E.; Kuentzmann P. Unsteady Motions in Combustion Chambers for Propulsion Systems. NATO Research and Technology Organization Neuilly-Sur-Seine: Paris, France, 2006. 664 pp.
7. Yash Pal, Ravi Kumar V. Physical and ballistic characterization of aluminum-loaded para?n hybrid rocket fuels. Energy & Fuels. American Chemical Society. 2017. V. 31. No. 9. Pp. 10133?10143.
https://doi.org/10.1021/acs.energyfuels.7b01636
8. Ganesan S., Chakravarthy S. R. Effect of acoustic pressure oscillations on burning rate augmentation of composite solid propellants at different initial grain temperatures. Combustion Science and Technology. 2023. https://doi.org/10.1080/00102202.2023.2248369
https://doi.org/10.1080/00102202.2023.2248369
9. Volkov V. T., Yagodnikov D. A. Study and Bench Tryout of Solid-Propellant Rocket Engines. Moscow: Bauman Moscow State Technical University, 2007. 296 pp.
10. Pylypenko O. V., Nikolayev O. D., Khoriak N. V., Bashliy I. D., Dolgopolov S. I. Dynamic processes in solid rocket motors and their interaction with rocket structure vibrations: the state of the art and current problems. Teh. Meh. 2023. No. 1. Pp. 3-13. (in Ukrainian).
https://doi.org/10.15407/itm2023.01.003
11. Prevost M., Vuillot F., Traineau J. C. Vortex shedding driven oscillations in subscale motors for the Ariane 5 MPS P230. 32nd AIAA/ASME/SAB/ASEE Joint Propulsion Conference and Exhibit, Lake Buena Vista, FL, July 1-3, 1996.
https://doi.org/10.2514/6.1996-3247
12. Taherinezhad R., Zarepour G. Theoretical, numerical and experimental investigation of vortex shedding in a novel sub-scaled motor. Journal of Applied Fluid Mechanics. 2019. V. 12. No. 4. Pp. 1319-1332.
https://doi.org/10.29252/jafm.12.04.29686
13. Bhutto A. A., Harijan K., Hussain M., Shah S.F., Kumar L. Numerical simulation of transient combustion and the acoustic environment of obstacle vortex-driven flow. Energies. 2022. V. 15. Iss. 16. 6079. 11 pp.
https://doi.org/10.3390/en15166079
14. Sanjeev Malhotra. On Combustion Instability in Solid Rocket Motors. Thesis of the dissertation for the degree of Doctor of Philosophy. California Institute of Technology Pasadena, California. 2004. 122 pp. URL: https://thesis.library.caltech.edu/1648/1/thesis.pdf
15. Ferretti V., Favini B., Cavallini E., Serraglia F., Giacinto M. D. Pressure oscillations simulation in P80 SRM ?rst stage VEGA launcher. San Diego. American Institute of Aeronautics and Astronautics. 2011. Pp. 102 - 116.
https://doi.org/10.2514/6.2011-6055
16. Dotson K. W., Sako B. H. Interaction between solid rocket motor internal flow and structure during flight. Journal of Propulsion and Power. 2007. V. 23. No. 1. Pp. 140 - 145.
https://doi.org/10.2514/1.20477
17. Glebov G. A., Vysotskaya S. A. On the design of solid-propellant rocket engines with the aim to eliminate combustion chamber working process instability. Vestnik Koncerna VKO "Almaz - Antey". 2017. No. 4. Pp. 63 - 72. (in Russian).
https://doi.org/10.38013/2542-0542-2017-4-63-72
18. Zhang Q., Wang N., Li J., Su W., Zhang Y. Effect of the head cavity on pressure oscillation suppression characteristics in large solid rocket motors. Science China. Technological Sciences. 2015. V. 58. No.7. Pp. 1250-1262.
https://doi.org/10.1007/s11431-015-5834-z
19. Kohnke P. Ansys Inc. Theory Manual. 001369. Twelfth Edition. - Canonsburg: SAS IP, 2001. 1266 pp.
20. Nikolayev O. D., Bashliy I. D. Assessment of thrust chamber stability margins to high-frequency oscillations based on mathematic modeling of coupled 'injector - rocket combustion chamber' dynamic system. Teh. Meh. 2022. No. 1. Pp. 3 - 17.
https://doi.org/10.15407/itm2022.01.003
21. Qin W. J., Xie M. Z., Jia M. et al. Large eddy simulation and proper orthogonal decomposition analysis of turbulent flows in a direct injection spark ignition engine: Cyclic variation and effect of valve lift. Sci. China Tech. Sci. 2014. V. 57. No. 3. Pp. 489-504.
https://doi.org/10.1007/s11431-014-5472-x
22. Nicoud F., Ducros F. Subgrid-scale stress modeling based on the square of the velocity gradient tensor. Flow Turbul. Combust. 1999. V. 62. No. 3. Pp. 183-200.
https://doi.org/10.1023/A:1009995426001
Copyright (©) 2023 Nikolayev O. D., Bashliy I. D., Khoriak N. V., Bondarenko S. H.
Copyright © 2014-2023 Technical mechanics
____________________________________________________________________________________________________________________________
|
GUIDE FOR AUTHORS
====================
Open Access Policy
====================
REGULATIONS
on the ethics of publications
====================
|